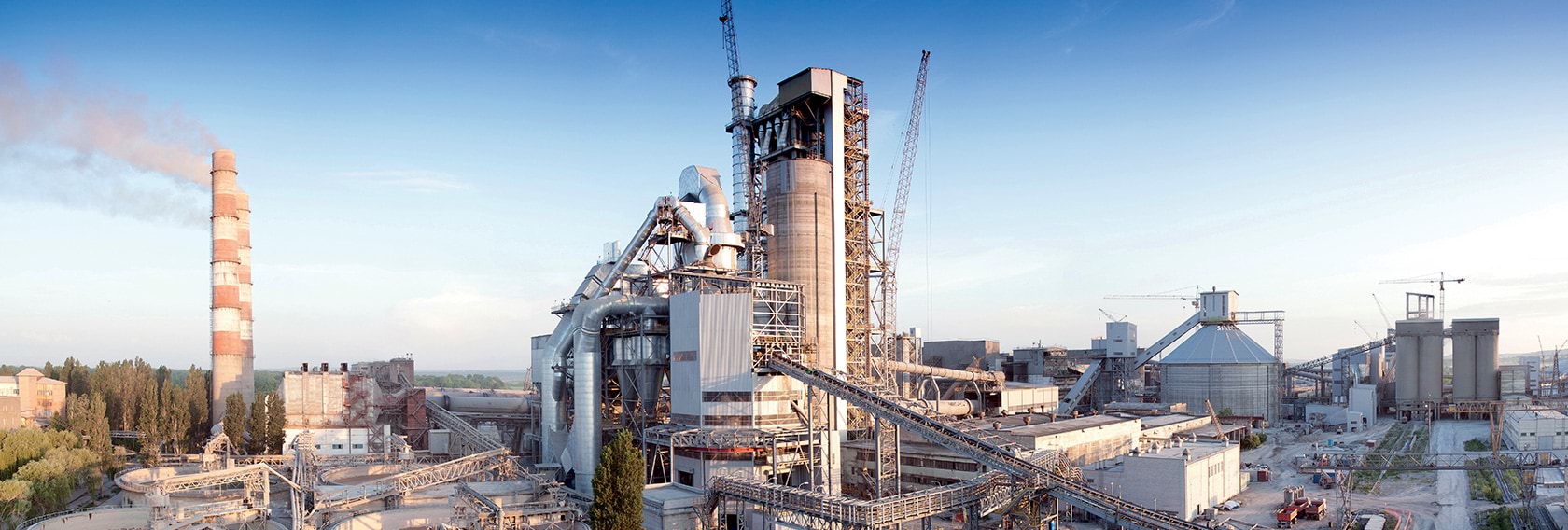
The cement industry and the most advanced analysis techniques
Concrete is still the most widely used construction material in the world.
The challenge for the cement industry is to continue to produce better performing cement while, at the same time, reduce costs and its impact on the environment.
How?
By using analysis techniques that enable its formulation to be analysed and improved and by providing constant, highly specialised technical support to the client.
We asked our experts to explain what they consider to be the most important techniques – from X-ray fluorescence to microscopy, a real world of Mapei solutions, all at your complete disposal.
In 2016 the global cement production was estimated at 4.6 billion tonnes, as mentioned, for example, in the Cembureau Activity Report 2016, available on the website of Cembureau (European Cement Association).
As usual, Asian countries account for three quarters of the total, with China being the biggest producer (2.3 billion tonnes). 4.6 billion tonnes would allow the batching of 15 billion m3 concrete, around 2 m3 for each person living on earth. Concrete is the most common building materials, thanks to its good mechanical performances, flexibility in use, durability and, last but not least, low cost. On the other hand, concrete and the Portland cement industry raise some environmental concerns, mainly related to the emission of greenhouse gases.
The manufacturing process of Portland cement is standardized and widely described in several publications. Raw materials (usually limestone and clays) are quarried, blended and ground in order to prepare the so-called “raw meal”. This is used as feeding for the kiln, where Portland clinker is produced. Clinker is then finely ground together with gypsum and secondary mineral additions in order to obtain Portland cement.
High CO2 emissions are associated to clinker production; hence, considering the stringent requirements in greenhouses gases reduction, blended cements with low clinker content (and several secondary cementitious materials – normally indicated as SCMs - such as limestone, pozzolan, fly ash, slag and so on) are the most widely used.
In the latest decades deep improvements have been made in the cement industry with the purpose of substituting traditional fuels (such as petcoke or natural gas, used to reach the high temperature needed for clinker production) with blends of different substances, coming from renewable resources or waste management.
Recent technologies for clinker production and the increased use of secondary cementitious materials promote different clinker chemistry and mineralogy studies, that should be taken into account in order to produce high quality cements. Hence, the optimization of cement performances is becoming a multi-disciplinary approach. As a matter of fact, it requires the correct management of physic-mechanical, mineralogical and microscopy data.
WHAT ARE THE MOST USEFUL ANALYTICAL TECHNIQUES TO STUDY CEMENTS?
When it comes to understand the difference between cement samples, the help of a well-equipped analytical laboratory is quite valuable. Big changes can be seen in reactivity and physical behavior, after the introduction of secondary fuels or the use of SCMs. Several techniques are helpful in assessing the influence of different parameters. In this article, we review the ones that, in our opinion, are the most important. The focus is on their significance as an analytical tool rather than on the scientific principles behind each of them. As a matter of fact, modern cement industry requires top level technical assistance. Hence, only top level companies, with a high specialization in cement chemistry, mineralogy and additives formulation, can be a reliable partner. All the techniques described here (and many more) are available in Mapei highly specialized Research&Development labs.
PARTICLE SIZE DISTRIBUTION
The particle size distribution (PSD) is a description of the number and dimensions of the particles that compose cement. It can be analysed in different ways. One of the most widely used methods is laser diffraction. Several instruments are available on the market, and the powder can be either dispersed in a flux of dry air or in a non-reactive liquid, e.g. ethanol. PSD can be used as a quality control parameter (for fineness checks, normally focused on a specific particle diameter) and as a means to assess the performance of the cement grinding process. This involves the calculation of the so-called “Tromp curve”, through which the optimization of the process can be determined. Grinding aids play a crucial role in the efficiency of the separator (the device that in modern cement grinding process allows obtaining a material with controlled fineness): a well-designed grinding aid can reduce the reject stream and minimize the bypass.
X-RAY FLUORESCENCE
X-Ray fluorescence (XRF) substituted the manual analysis of clinker and other components in most modern cement plant laboratories.
It is a quick, reliable and relatively easy method to determine the chemical composition of a sample, allowing to check constancy of composition, the presence of minor elements, etc.
QUANTITATIVE X-RAY DIFFRACTION
Quantitative X-Ray diffraction (XRD with Rietveld refinement) is an excellent tool to evaluate the mineral phases in materials. It reveals important features of cement minerals, including the real amount of individual phases, the types of calcium sulfate, the polymorphism of calcium silicates, aluminates and ferrite, and the presence of free lime, portlandite, and minor but important alkali sulfate phases. The information provides guidance to the cement processing condition. More importantly, it is a powerful tool for troubleshooting performance issues in the field.
THERMOGRAVIMETRIC ANALYSIS
In thermogravimetric analysis, a sample of material is heated from a very low temperature (e.g. -40°C) up to high temperature (e.g. 1000°C), and all weight changes are recorded. This allows to assess several characteristics of finished cement, namely pre-hydration, calcite content, dehydrate and hemihydrate calcium sulfate water loss, syngenite presence, and possibly others, as a quantitative determination. Normally this technique is coupled with Differential Thermal Analysis (DTA), that adds information relevant to the heat exchange (showing if the transformations are exothermic or endothermic). TGA and DTA can be very helpful in diagnosing many issues reagarding cement quality, storage flowability and prehydration.
DIFFERENTIAL SCANNING CALORIMETRY
Differential Scanning Calorimetry (DSC) is similar to TGA-DTA analysis, but instead of weight changes, heat transfer is recorded and measured. This allows to quantify the energy associated to phenomena triggered by temperature change. In cement analysis, DSC is particularly accurate in the determination of gypsum dehydration rate, providing guidance on mill operations. In general, the gypsum dehydration rate influences cement performances in several ways: an incorrect gypsum dehydration can negatively affect the normal hydration profile of cement, change its hydration kinetics, modify the flowability of the cement mortar and the concrete slump.
ISOTHERMAL AND ADIABATIC CALORIMETRY
The isothermal calorimetry is an excellent tool to determine cement hydration kinetics and monitor the cement hydration process. The hydration profiles from calorimetric tests can help to predict the sulfate balance, the strength development potential and the cement compatibility with concrete admixtures. Isothermal calorimetry is a very good technique to assess differences in hydration profile provided by cement additives used as performance enhancers.
Adiabatic calorimetry is complementary to the isothermal calorimetry. Hydration kinetics are studied at the temperatures generated by the heat of reaction of cement, with no correction as in isothermal experiments. Concrete, or mortar, specimens are used, to better simulate a real-world situation. This allows to troubleshoot concrete performances more effectively, and it is a useful method to study the interactions between cement additives and concrete admixtures.
SEM AND OPTICAL MICROSCOPY
Burning, cooling and process conditions of the kiln at cement plants are directly responsible for the reactivity of the mineral phases of the clinker and the quality of cement. Optical microscope analysis directly reveals the burning process of the clinker, including the size of the crystals and their distribution in the matrix, kiln atmosphere and reduced condition, burning temperature, and primary and secondary cooling rates. Preparation of the clinker sample (see Figure 1) includes selecting a significant specimen, crushing it and sieving it to select an homogenous crystal size, embedding it in resin, polishing with a suitable equipment, and observing it in reflected light.
The scanning Electron Microscope (SEM) is a very powerful instrument that can be used to directly observe the shape and dimensional distribution of cement and raw meal particles. At the same time, with EDX spectroscopy, it is possible to detect the chemical composition of particles or areas of the sample. The technique has proved itself very useful in troubleshooting raw meal (the blend of limestone and clay used for clinker production) issues and their combinability in the kiln. Through observation of the particles of cements, interesting information on the morphology and physical behavior can be obtained (see figures 2 and 3).
Crossing the information coming from all these analytical techniques can provide the difference in being able to meet the market’s requirements for a modern cement plant, and to select the right cement additive to help a plant to do it in the most economical way.
For any further information , please consult our dedicated online section on C-ADD Mapei Division
Paolo Forni, Matteo Magistri. Cement Additives Division, Mapei SpA